![]() |
Among the most basic questions in quantum electronic transport is what happens when a quantum conductor is driven by an ac voltage. We recently addressed theoretically what happens when this ac voltage is itself truly quantum mechanical in nature (being described by for example a squeezed state or a Fock state). This issue has been recently addressed theoretically by a team from Laboratoire de Physique des Solides within the framework of the project QEDMicroscOP.
|
One of the most important achievements in quantum optics over the past decades was the ability to trap microwaves photons in a superconducting cavity and to control their interaction with Rydberg atoms crossing the cavity. Pioneering experiments of this type were achieved in the groups of D. Wineland and of S. Haroche, the former at NIST, University of Colorado at Bolder, the latter at the Ecole Normale Supérieure. This field belongs to the domain of research called “Cavity Quantum Electrodynamics’’ (CQED). Similar physics was observed a decade ago when Rydberg atoms are replaced by a two-level solid state system, often called an artificial atom, capacitively coupled to a single mode of the microwave cavity. Swift progress have been realized in that direction mainly because these systems offer a full electronic and therefore easier control. In addition, the microwave photons in the cavities can be generated and detected by “standard” microwave electronics. Thus, superconducting quantum circuits have partially merged quantum optics and quantum electronics.
The impressive development of CQED in superconducting circuits and of non-linear optics during the last decade has recently enabled to generate quantum states of the electromagnetic field which have no classical equivalent such as the Fock state or the Schrödinger’s cat state. A superconducting resonator driven into a Fock state is characterized by a constant number of microwave photons sustained in the cavity while a Schrödinger’s cat state is a coherent superposition of two macroscopic states, for example, two dephased coherent states in a microwave cavity. One may wonder which kind of experimental scheme do we have to use to reveal the quantum nature of such particular photons. In this work, we made use of the ability to coherently couple microwave photons in low-loss superconducting cavities to quantum electronic conductors to detect electrically an optical quantum state. Concretely, we have studied theoretically the new physics emerging when a quantum electronic conductor is exposed to non-classical microwave radiation. From an electronic viewpoint, this corresponds to a non-trivial ac voltage across the quantum conductor.
Experimental systems exist that could realize such a situation: by coupling a tunnel junction to a superconducting microwave cavity. We show that the current of the conductor has signatures that directly reflect the non-classical nature of the driving microwaves. In that sense, a measure of an electric current can be used as a detector of the quantum nature of the microwave optical electric field. Such feature is illustrated in the figure where we illustrate a Fock state with two microwave photons in the cavity which entails a strongly non-monotonic dependence of the differential conductance of the tunnel junction with the bias voltage. Moreover, the transport is naturally associated with quasi-probabilities that can become negative.
Figure : Differential conductance dI/dV versus dc bias voltage V for a tunnel junction coupled to a microwave cavity initially prepared in the Fock state n=2. The striking signature of a non-classical state here is the strongly non-monotonic dependence of the first few conductance plateaus on voltage; in particular, the height of the second plateau (h2) is smaller than the first (h1). This is in one to one correspondence in the Fock state with exactly two photons in the cavity.
Référence : Photon-assisted tunnelling with nonclassical light, J. -R. Souquet, M. J. Woolley, J. Gabelli, P. Simon and A. A. Clerk, Nature Communications, 5, 5562 (2014).
Results achieved in the framework of the project ” Quantum electrodynamics of a quantum conductor : from microwaves to optical photons” (QEDMicroscOP 2013-2016) funded by the theme 1 of the LabEx PALM and carried out by Pascal Simon (LPS).
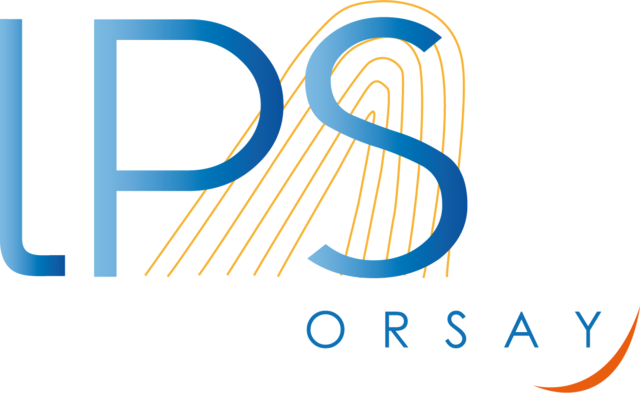
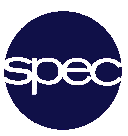